Quantum computing represents a technological paradigm shift, providing a new approach to processing information and solving previously inaccessible problems to classical computers.
The strength of quantum computing stems from its ability to exploit the unique properties of quantum mechanics, which has the potential to revolutionize fields such as cryptography, optimization, materials science, and drug discovery.
Quantum bits, or qubits, are at the heart of quantum computing. These fundamental building blocks allow quantum computers to process and store data in ways that traditional bits cannot. Because of their long coherence times, high-fidelity operations, and scalability potential.
Trapped ion qubits have emerged as promising candidates among the various physical platforms for realizing qubits.
Table of Contents
A Primer on Trapped Ion Qubits
Because of their distinct advantages and capabilities, trapped ion qubits have emerged as one of the leading platforms for quantum computing. In this primer, we will discuss the fundamental concepts and properties of trapped ion qubits, their benefits, challenges, and the underlying principles of their operation.
What is the difference between trapped ions and hyperfine qubits?
Trapped ions are charged atomic particles held together in space by electromagnetic fields. These trap ions can be precisely manipulated and controlled using laser beams or microwave radiation, making them an appealing platform for quantum computing. Internal atomic states within these trapped ions can encode quantum information, producing hyperfine qubits.
The hyperfine structure of atomic energy levels is used to create hyperfine qubits. This is caused by the interaction of the magnetic moments of the atomic nucleus and the electrons that orbit it. We can represent quantum information in the form of |0> and |1> states by using two specific hyperfine states as the basis for the qubit.
Internal hyperfine states of trapped ions can be manipulated using laser or microwave radiation, allowing the implementation of quantum gates and the execution of quantum algorithms.
Advantages of trapped ion qubits over other types of qubits
Trapped ion qubits have several advantages over other types of qubits, such as superconducting, topological, or photonic qubits, making them a promising candidate for quantum computing:
- Trapped ions have longer coherence times than other qubit types, meaning they can maintain their quantum states for relatively long periods without decoherence. This enables more complex quantum operations to be executed with greater fidelity.
- Trapped ions allow for precise control and manipulation of quantum states, resulting in high-fidelity single-qubit and two-qubit gate operations. This precision is critical for running complex quantum algorithms and avoiding errors.
- Trapped ions can be scaled up using ion-trap arrays or microfabricated ion traps, possibly building large-scale quantum computing systems.
- Addressability on an individual basis trapped ions can be spatially separated and individually addressed using focused laser beams, allowing specific qubits to be manipulated without affecting the rest of the system.
Trapped ion challenges and limitations
Despite their promising characteristics, trapped ion qubits face several challenges and limitations that must be addressed before they can reach their full potential in quantum computing:
- Although trapped ion qubits have longer coherence times than other qubits, decoherence remains difficult. External magnetic fields, temperature fluctuations, and laser intensity variations can all affect quantum states, limiting the performance of quantum operations.
- Precisely controlling trapped ion qubits frequently necessitates intricate laser setups or microwave systems, which can be challenging to implement and maintain. The system’s complexity grows as the number of qubits increases, posing a scalability challenge.
- Gate velocity in trapped ion systems. The speed of quantum gates is typically slower than in other qubit types, such as superconducting qubits. The slower gate speed of trapped ion-based quantum computers may limit their overall computational power.
- Engineering difficulties scaling trapped ion qubits to larger systems necessitates the development of sophisticated ion trap architectures and control systems, both of which pose significant engineering challenges.
With their distinct advantages and scalability potential, trapped ion qubits represent a promising avenue for quantum computing. On the other hand, addressing their challenges and limitations will be critical to realizing their full potential and harnessing the power of quantum computing in the future.
Join our newsletter, tip of the spear
Hyperfine Qubit Manipulation
The manipulation of hyperfine qubits is critical in developing quantum computing using trapped ion qubits. This section will review the fundamentals of hyperfine qubit manipulation, such as single-qubit rotations, two-qubit operations, and qubit preparation and measurement procedures.
Single-qubit rotations
Single-qubit rotations are fundamental operations in quantum computing that involve changing a single qubit’s excited state without affecting the other qubits’ excited states in the system. Single-qubit rotations in the context of trapped ion hyperfine qubits can be accomplished by using laser or microwave radiation to drive transitions between the two selected hyperfine states representing the |0> and |1> states of the qubit.
A precisely tuned and controlled electromagnetic field is applied to the trapped ion to perform single-qubit rotations of multiple ions. The field’s frequency, phase, and duration determine the rotation’s type and extent, allowing manipulation of the qubit’s state.
These rotations can be described mathematically using the Bloch sphere representation, where a point on the surface of a sphere represents the qubit’s state.
Two-qubit operations and entanglement
Two-qubit operations are required for the development of quantum algorithms and the implementation of error correction techniques. These operations involve simultaneously manipulating the states of two qubits.
They can cause entanglement, a uniquely quantum phenomenon in which the conditions of two qubits become strongly correlated to the point where the form of one cannot be described independently of the state of the other.
Various techniques, such as the Cirac-Zoller gate, the Mlmer-Srensen gate, and spin-dependent optical forces, can perform two-qubit operations in trapped ion systems. These methods involve coupling the qubits’ internal states to the motional degrees of freedom of the ions, which are then used to mediate interactions between target ions and the qubits.
Entangling operations can be performed by carefully controlling the strength and duration of the exchange, resulting in the creation of two-qubit quantum gates, such as the Controlled-NOT (CNOT) gate or the controlled-phase gate.
Qubit preparation and measurement
Qubit preparation and measurement are critical steps in quantum computing that involve putting qubits into a known state and deriving the results of quantum operations. These processes can be carried out precisely and efficiently using laser-induced fluorescence techniques in trapped ion systems.
The ion is optically pumped into a specific hyperfine ground state, which serves as the initial quantum operations state for quantum computation, to prepare a trapped ion hyperfine qubit. This preparation usually yields high fidelity, providing a reliable starting point for subsequent analyses.
Quantum state-selective fluorescence is a method for measuring the state of atomic ions trapped in a hyperfine qubit. Using resonant laser radiation, the trapped ion will only emit fluorescence in a specific hyperfine state. The qubit’s state can be easily deduced by detecting the emitted photons.
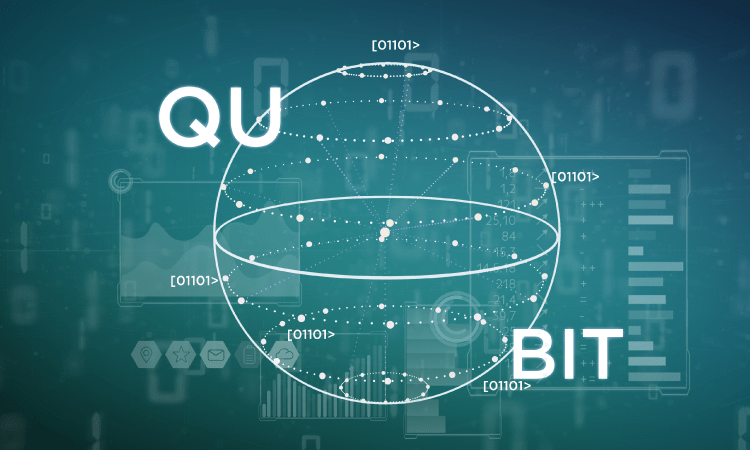
Gate Schemes for Trapped Ion Qubits
In quantum computing, gate schemes are critical for manipulating and controlling trapped ion qubits. This section will discuss the advantages and disadvantages of several important gate schemes, including the Cirac-Zoller Gate, Sørensen-Mølmer-Solano Gate, Raman Carrier Operations-Based Gate, and Spin-Dependent Optical Force-Based Gate.
Cirac-Zoller Gate
One of the earliest proposals for implementing two-qubit quantum gates in trapped ion systems was the Cirac-Zoller gate. It entails using laser pulses to couple the internal states of the qubits with the motional degrees of freedom of the ions, resulting in entanglement. The main advantage of the gate is its simplicity and ability to create entangling gates such as the CNOT gate.
However, precise emotional state control, individual ion addressing via laser-beam focusing, and an additional internal state are required. Because of these requirements, it is more challenging to implement in practice than other gate schemes.
Sørensen-Mølmer-Solano Gate
The Sørensen-Mølmer-Solano gate is an alternative to the Cirac-Zoller gate with several advantages. It does not require individual trapped ion quantum computer addressing, can be carried out in one step, does not require an additional internal state, and does not need precise control of the emotional state ion trap quantum computer as long as the Lamb-Dicke limit is satisfied.
This gate scheme has been successfully implemented in experiments, demonstrating its practicality and effectiveness for trapped ion quantum computing.
Raman Carrier Operations-Based Gate
The Raman carrier operations-based gate is a Cirac-Zoller gate variant that uses Raman transitions to achieve single-qubit rotations. By adjusting the Lamb-Dicke parameter, the emotional state affects the carrier operations, resulting in a -phase gate with a single pulse of light.
This method is more robust and efficient than the Cirac-Zoller gate because it requires fewer steps, does not require an auxiliary internal state, and is immune to Stark shifts caused by off-resonant sideband couplings.
Spin-Dependent Optical Force-Based Gate
Spin-dependent optical force-based gates couple hyperfine qubits with ion motion by utilizing spin-dependent forces generated by laser beams. They typically provide gates that do not change the qubit state, such as phase gates, and can provide better isolation from qubit rotation errors.
Meaning that gate speeds for this method may be faster than for other entrances. However, these gates only apply to magnetically sensitive hyperfine qubit states, making the qubits more vulnerable to fluctuating magnetic fields.
Comparison of gate schemes: advantages and disadvantages
Each of the aforementioned gate schemes has distinct advantages and disadvantages. The Cirac-Zoller gate is simple but necessitates precise control and additional internal states. The Sørensen-Mølmer-Solano gate is more practical and efficient, but it may not be appropriate for all applications.
The Raman carrier operations-based entrance is more robust and efficient but may be less versatile. Finally, the spin-dependent optical force-based gate operates at high speeds but is limited to magnetically sensitive states.
Experimentation and Demonstrations
Numerous milestones and breakthroughs have occurred on the path of trapped ion qubits from a theoretical concept to experimental realization. This section will cover major experimental advances and demonstrations in trapped ion qubit research, as well as remaining challenges and future research directions.
Milestones in trapped ion qubit experiments
Since their inception, trapped ion qubits have seen significant experimental progress. Among the most notable achievements are:
- In 1995, a CNOT gate between the motion and internal state of a single trapped ion was demonstrated by implementing the essential elements of the original Cirac-Zoller gate.
- In 2003, the full Cirac-Zoller gate on two ions was implemented with approximately 70% fidelity.
- In 1999, the Sørensen-Mølmer-Solano gate was proposed as an alternative gate scheme, later demonstrated by the NIST group in the early 2000s.
- In 2002, a Raman carrier-based variation of the Cirac-Zoller gate was demonstrated.
- In 2003, the NIST group demonstrated a -phase gate based on a qubit state-dependent force between two trapped ion qubits, achieving a Bell state with 97% fidelity.
Recent advancements and breakthroughs
Several significant advances in the electric field of trapped ion qubit research have occurred in recent years:
- Better control of environmental factors such as magnetic field fluctuations and ion trap fabrication has improved qubit coherence times.
- Optimizing laser parameters, lowering motional heating rates, and implementing error correction techniques all help to improve gate fidelities.
- Miniaturization and integration of ion traps are progressing, paving the way for scalable trapped ion quantum computers.
- Creating new, more efficient quantum error correction gate schemes and protocols improves the overall performance of trapped ion quantum computers.
- Multi-qubit entanglement, quantum error correction codes, and small-scale quantum algorithms using trapped ion qubits are all demonstrated.
Remaining challenges and future research directions
Despite significant progress, there are several challenges and open questions in trapped ion qubit research:
- One of the most difficult challenges is scaling trapped ion quantum computers to many qubits while maintaining high gate fidelities and coherence times.
- Error rates: While gate reliabilities have improved, they still need to be reduced further to meet the requirements of fault-tolerant quantum computing.
- Decoherence: Coherence times must be improved further to implement more complex quantum algorithms and error correction protocols.
- System integration: For large-scale trapped ion quantum computers to be realized, integrated systems for laser control, ion manipulation, and readout must be developed.
- Novel gate schemes and architectures: For trapped ion quantum computing, it will be critical to investigate new systems and architectures that can improve gate fidelities and speeds.
Addressing these challenges and exploring new techniques will be critical in realizing the full potential of trapped ion quantum computers as trapped ion research advances.
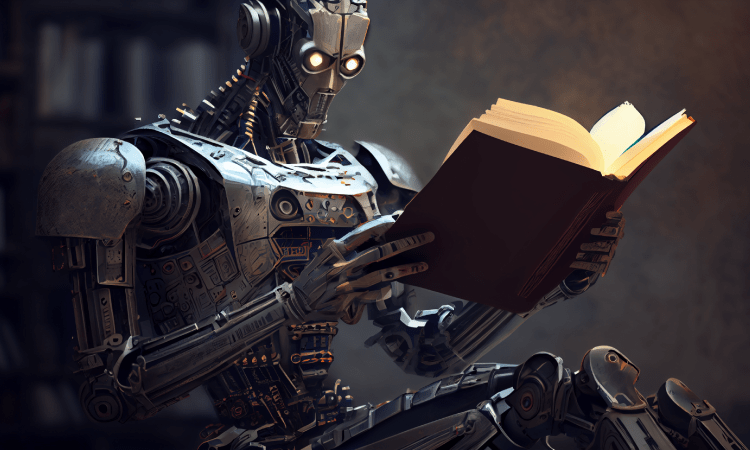
Applications and Future Perspectives
Trapped ion qubits have the potential to play a significant role in the advancement of quantum computing as it evolves. In this section, we will look at the various applications of trapped ion qubits in quantum computing, their role in the future of quantum technology, and the importance of interdisciplinary collaboration in pushing the boundaries of this emerging field.
Potential applications of trapped ion qubits in quantum computing
Trapped ion qubits hold great promise for various quantum computing applications, including.
Trapped ions for quantum simulation can simulate complex quantum systems and provide insights into many-body quantum problems in condensed matter physics, quantum chemistry, and materials science.
Cryptography quantum key distribution (QKD) protocols can use trapped ion qubits for secure communication, providing unprecedented security against eavesdropping.
Optimization issues Trapped ion quantum computers can solve complex optimization problems faster than classical computers, with potential logistics, finance, and machine learning applications.
Error correction at the quantum level Trapped ion qubits can be used in quantum error correction codes, which are required to construct fault-tolerant quantum computers.
Algorithms for quantum computing Trapped ion qubits can implement functional quantum computer algorithms like Shor’s and Grover’s, potentially transforming fields like cryptography, search, and optimization.
The role of trapped ion qubits in the future of quantum technology
Trapped ion qubits, which have long coherence times and high gate fidelities, are expected to play an essential role in the future of quantum technology. Some possible parts include.
Along with other technologies, such as superconducting qubits and topological qubits, we are establishing trapped ion quantum computers as one of the leading platforms for quantum computing.
It is working on hybrid quantum systems that combine trapped ion qubits with other quantum technologies, which could lead to new functionalities and improved performance of quantum system.
Assisting in developing new gate schemes, quantum error correction protocols, functional quantum computers, and quantum algorithms.
Collaboration and interdisciplinary research for advancements
Collaboration and interdisciplinary research will likely be required to advance trapped ion qubit technology.
Collaboration between atomic, molecular, and optical (AMO) physicists, quantum information scientists, and condensed matter physicists is critical for refining understanding of trapped ion systems and improving experimental techniques.
Engineering collaboration with electrical and mechanical engineers is critical for developing integrated systems that can scale up trapped ion quantum computers, such as ion traps, laser systems, and control electronics.
Working with quantum computer scientists and theorists in computer science will be required to develop new quantum algorithms, error correction codes, and optimization techniques tailored for trapped ion qubits.
Materials science collaboration can help improve the performance of ion traps and other components, allowing for faster coherence times and higher gate reliability.
Industry collaboration and partnerships will be required to facilitate the commercialization and widespread adoption of trapped ion qubit technology in various applications.
The trapped ion qubit community can overcome existing challenges and pave the way for practical, large-scale quantum computers by encouraging collaboration and interdisciplinary research.
Charting the Quantum Horizons: A Final Look
Because of their long coherence times, high gate fidelities, and precise control, trapped ion qubits have emerged as a promising platform for quantum computing. Their potential applications include quantum simulation, cryptography, optimization problems, quantum error correction, and quantum algorithms, making them an essential technology for leveraging the power of quantum computing.
It is critical to continue research and development in this field to realize the potential of trapped ion qubits fully. Efforts should be focused on improving experimental techniques, developing novel gate schemes, and overcoming limitations such as scalability and noise susceptibility of optical qubits.
Furthermore, research should focus on developing advanced quantum algorithms and error correction protocols for trapped ion qubits, which will be required for building practical, large-scale quantum computers.
Breakthroughs and innovations in trapped ion qubits will almost certainly necessitate interdisciplinary collaboration across multiple disciplines, including physics, engineering, computer science, and materials science. Such associations will enable the development of integrated systems, novel algorithms, and improved materials to advance trapped ion qubit technology.
Partnerships with industry will also aid in the commercialization and widespread adoption of trapped ion qubit technology in various applications, ultimately contributing to the development and success of quantum computing. The trapped ion qubit community can overcome existing challenges and realize the immense potential of quantum computing by collaborating across disciplines.
What are trapped ion qubits?
Individual ions are trapped using electromagnetic fields and manipulated using laser beams to form trapped ion qubits, which are used in quantum computing. Because of their long coherence times and high-fidelity operations, these qubits use the same single trapped ions’ hyperfine energy levels to store and process quantum information, making them a promising platform for quantum computing.
What is trapped ion quantum computing?
Trapped ion quantum computing is a technique that uses trapped ions as qubits to perform quantum operations and process data. Ions are confined using electromagnetic fields in this method. Their quantum states are manipulated using precisely controlled laser beams, allowing for high-fidelity quantum gates and long coherence times in robust quantum computations.
What are the advantages of trapped ion qubits?
Long coherence times, high-fidelity quantum gate operations, and individual qubit addressing via precise laser control are all advantages of trapped ion qubits. Furthermore, they enable robust and long-distance qubit-qubit interactions, making them a promising platform for scalable quantum computing systems.
Which is better superconducting qubits or trapped ions?
Choosing between superconducting and trapped ion qubits is difficult because each has advantages and disadvantages. Superconducting qubits are more compatible with existing semiconductor fabrication techniques and have faster gate operations. Trapped ion qubits, on the other hand, have higher-fidelity gate operations and longer coherence times. Specific application requirements and the priorities of the quantum computing system under development influence the decision between the two.